The
non-scientific mind has the most ridiculous ideas of the precision of
laboratory work, and would be much surprised to learn that, excepting
electrical measurements, the bulk of it does not exceed the precision of an upholsterer who comes to measure a window for a pair of curtains.
—Charles S. Peirce (1908)
No doubt Charles Sanders Peirce (1839–1914) was exaggerating somewhat. But when it came to metrological matters, Peirce (pronounced purse)
knew what he was talking about. Best known as a logician,
mathematician, and America’s most original philosopher (a founder of the
philosophical school known as pragmatism), he was also a leading
scientist. 1 1.
V. Lenzen, Proc. Am. Phil. Soc. 109, 29 (1965). Peirce made precision measurements, and improved techniques for making them. His work helped remove American metrology from under the British shadow and usher in an American tradition.
Peirce was the first to experimentally tie a unit, the meter, to an absolute standard, the wavelength of a spectral line.
For several reasons, that contribution has not received much attention.
First, he never finished it to his satisfaction and left only
fragmentary reports in his 12 000 published pages and 80 000 pages of
handwritten notes and letters—mostly on logic, mathematics, science,
and philosophy. Second, Peirce’s idea was almost immediately taken up
and significantly improved by Albert Michelson, using the interferometer that he and Edward Morley had built to measure
ether drift. Finally, Peirce’s chaotic professional and personal life
has hindered a comprehensive assessment of his contributions.
Peirce was a prolific and perpetually overextended polymath, but his personal life also contributed to his career
woes. He suffered from several illnesses, including an excruciating
inflammation of facial nerves now called trigeminal neuralgia and severe
mood swings that today would be diagnosed as bipolar disorder. Recourse
to the common treatments of the day, including ether, opium, and
cocaine, compounded the social challenges created by the diseases.
Peirce nearly landed professorships at Harvard University and the Johns
Hopkins University, but conflicts with supporters and benefactors
wrecked his chances. His fractious personality is so difficult to
encompass that Joseph Brent, author of the only full-length Peirce biography, threw up his hands and resorted to a laundry list of psychopathologies:
On the manic side he exhibited driven, paranoid, and
impulsive actions; extreme insomnia; manic grandiosity and
visionary expansiveness; hypersexuality; extraordinary energy; and
irrational financial dealings, including compulsive extravagance
and disastrous investments. On the depressive side, he
exhibited severely melancholic or depressive states
characterized by suicidal feelings or flatness of mood, which were
accompanied by inertness of mind, inability to feel emotion,
and an unbearable sense of futility. 2 2.
J. Brent, Charles Sanders Peirce: A Life, Indiana U. Press, Bloomington (1998).
Some
future biographer may be able to integrate such behaviors with Peirce’s
life of multitasking, extraordinary productivity, and original insights
to produce a more comprehensive and positive portrait of this
remarkable American genius. Peirce indeed had an original personality,
but he had an even more original mind.
Peirce
had an enviable start. He was born to an elite family in Cambridge,
Massachusetts. He was handsome and articulate, collected friends in high
places—and then spent his career
squandering those advantages. Peirce’s father, Benjamin (1809–80), was a
professor of mathematics and astronomy at Harvard and an administrator
in the US Coast Survey. Grooming his son for science,
Benjamin sent Charles to private school and Harvard, from which the boy
graduated in 1859 at the age of 19. Then he attended the Lawrence
Scientific School, Harvard’s early graduate program in engineering and the sciences (which Benjamin had helped to found), and received a Master of Science degree in chemistry summa cum laude in 1863.
Charles
was fascinated by logic, viewed himself as a logician, and yearned to
work full-time on the subject. But Benjamin, wishing to promote his
son’s education and career, arranged a series of scientific apprenticeships. One was with the Coast Survey. Established in 1807, the Survey was the US
government’s preeminent scientific agency. In the 1860s it had a high
profile because of work in connection with the purchase of Alaska from
Russia and with the proposed purchase of Greenland and Iceland from
Denmark. Charles’s first official position at the Survey came in 1861,
at the beginning of the Civil War, when Benjamin lost an important
computational aide and got his son appointed as the replacement. In 1867
Benjamin became superintendent of the Survey, ran the office from
Cambridge, and appointed his son first as an aide and then, in 1872, as
an assistant directly beneath him in rank.
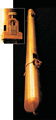
A gravimetric pendulum,
one of several designed and used by Charles Peirce in the 1870s and
1880s to measure the acceleration of gravity at various locations. The
brass pendulum is about 1.6 meters long. Its cylindrical symmetry
facilitated the calculation of corrections for oscillation in a viscous
medium.
(Courtesy of the Smithsonian Institution.)STEFAN KABEN
Meanwhile, Benjamin arranged a second apprenticeship for his son at the Harvard College Observatory, whose director, Joseph Winlock, was a close friend. Spectroscopy was in its infancy, and the observatory acquired its first spectroscope
in 1867. Charles helped Winlock with observations of stellar spectra
and became Winlock’s assistant. In that capacity, Peirce was sent to
carry out measurements of two solar eclipses. Stationed in Kentucky during the eclipse
of 1869, he became one of the first to observe the spectrum of argon. A
year later he and his wife Melusina (“Zina”), a prominent feminist,
observed another eclipse in Sicily. He also embarked on an ambitious attempt to use the relative brightnesses of stars to determine the shape of the galaxy.
In 1872, the year Peirce ended his connection with the observatory,
he wrote his mother about his overstretched work habits: “On clear
nights I observe with the photometer; on cloudy nights I write my book
on logic which the world has been so long & so anxiously expecting.”
The logic book, like so many other Peirce projects, was never
completed. The observations resulted in Photometric Researches
(1878), the only book published in his lifetime. Its impact, however,
was blunted by a three-year publication delay due to his overcommitments
and quarrels with publishers and colleagues.
Also
in 1872, Peirce was a founding member of the Metaphysical Club, a
discussion group of prominent intellects of the day, whose members
included philosopher William James and jurist Oliver Wendell Holmes Jr.
In the following years the group originated the basic concepts of
pragmatism as a philosophical movement, according to which meaning and
truth are to be sought in the practical consequences of concepts and
beliefs. As James once put it: “Truth is what works.”
Thus by 1872 Peirce had made a good start at a career: high-profile positions, excellent prospects, and influential friends. He still considered himself a logician. But career
prospects in logic were few, and he saw his scientific work as
enriching his ideas about logic. Among other things it left him with an
understanding of the importance and limitations of precision.
Two kinds of precision research in particular prepared Peirce for the measurement that tied the meter to optical wavelengths. One, from his observatory work, was photometrics, which involved spectroscopes, diffraction gratings, and wavelength measurements. The other preparation, which he was about to get at the Survey, was gravimetrics, which involved the theory and practice of pendulums and their calibration.
International interest in precision was cresting. Metrology
advanced swiftly in the late 19th century with the second phase of the
Industrial Revolution. Peirce’s remark about upholsterers was accurate;
electrical measurements
were the vanguard. Beginning in the 1840s, a developing and expanding
telegraph industry, particularly in Britain, drove the establishment of
electrical standards and of institutions needed to set and supervise
them. 3 3.
I. Morus, When Physics Became King, U. Chicago Press, Chicago (2005).
The 1851 Great Exhibition of the Works of Industry of All Nations at
London’s Crystal Palace, the first great international exposition to
feature industrial machinery, was a showcase for interchangeable
production methods. The exhibition provided a major stimulus for greater
international cooperation in setting measurement units and standards.
Another
driver was the increasing international importance of geodesy—one of
whose principal activities was gravimetrics, the precision measurement of gravitational variations over Earth’s surface. The initial meeting of the International Geodetic Association (IGA), the first international scientific association, was held in Berlin in 1864. A metrology conference in Paris in 1872 sought to create an international bureau of weights and measures.
The outcome was the 1875 treaty establishing such an institution and
initiating the production of new metrological artifacts such as the
standard meter. The institution, the Bureau International des Poids et
Mesures (BIPM), is still located in Sèvres near Paris.
Michelson’s comment that “our future discoveries must be looked for in the sixth place of decimals,” in his 1902 book Light Waves and Their Uses,
is only the most frequently cited of similar remarks by scientists of
the day. Peirce shared that sentiment. In 1878, when he was under
consideration, along with Henry Rowland, to chair the physics department
at the new Johns Hopkins University, Peirce wrote to the university’s
president that “the new phenomena which now remain to be discovered are
probably only of a secondary importance” and that progress requires “the
salutary severity of exact science.”
Such
late-19th-century remarks are often interpreted as a naive lament: The
era of great scientific breakthroughs is over, our picture of nature is
nearing completion, and the work that remains is dry number chasing. But
the situation was more complex. Not only were practical demands for
precision mounting, but many researchers
found its pursuit exciting. They thought it might lead to otherwise
inaccessible truths, and even—in Victorian culture—saw it as an ethical
good. 4 4.
S. Schaffer, in Victorian Science in Context, B. Lightman, ed., U. Chicago Press, Chicago (1997), p. 438.
In Michelson’s next sentence after the one quoted in the previous
paragraph, he wrote, “It follows that every means which facilitates
accuracy in measurement is a possible factor in a future discovery.”
Thus when physicists
in the late 19th century articulated their goal as a search for
increasing precision, that did not imply a genteel slackening of pace.
If anything, the pace was intensifying—reflecting practical urgency,
national interest, theoretical
significance, and moral value. The expectation that future discoveries
would lie in decimal places was soon vindicated by the discovery of the
quantum in measurements of blackbody radiation made at a metrological institute and the confirmation of general relativity in tiny deviations of starlight.
Allied
with the late-19th-century quest for precision was a search for a
universal and absolute standard of length. Unsatisfactory attempts had
been made to tie the meter to a fraction of Earth’s circumference and to
the length of a so-called seconds pendulum—one whose period is
precisely two seconds. Those failures led some to propose that the meter
eventually could be tied to light. For instance, at the beginning of
his magnum opus, A Treatise on Electricity and Magnetism (1873), James Clerk Maxwell remarked that “in the present state of science
the most universal standard of length which we could assume would be
the wave length in vacuum of a particular kind of light, emitted by some
widely diffused substance such as sodium, which has well-defined lines in its spectrum.” In his characteristically wry manner, Maxwell
added that such a standard “would be independent of any changes in the
dimensions of the earth, and should be adopted by those who expect their
writings to be more permanent than that body.”
Peirce’s route to a new standard began in 1872, when Julius Hilgard, the head of the Survey’s Washington, DC, office, left for Paris to represent the US at the metrology
conference. In Hilgard’s absence, Superintendent Peirce appointed his
son Charles to be acting head. One of Charles’s duties was to head the
Survey’s Office of Weights and Measures in Washington. That duty brought him into contact with the growing international metrological community.
Benjamin,
meanwhile, was further elevating the Coast Survey’s profile. In 1871 he
convinced Congress to mandate a transcontinental geodetic survey along the 39th parallel, connecting surveys already taken along both coasts. Geodesy would rapidly become the Survey’s chief work, and the agency would soon be renamed the Coast and Geodetic
Survey. Pendulums were its principal gravimetric instruments. After
Hilgard’s return, Benjamin put Charles in charge of the Survey’s
pendulum research. That work involved the use of precision length
standards to calibrate the pendulums.
The
IGA had organized a network of gravimetric surveys and by 1872 had
chosen as its instrument a reversible pendulum designed by German astronomer
Friedrich Bessel and manufactured by a Hamburg firm of instrument
makers. Peirce ordered one, but its completion was delayed while the
firm filled orders for instruments to measure the 1874 transit of Venus. When the instrument was completed in 1875, Peirce traveled to Europe to pick it up.
During
his European trip, Peirce met scientists and scholars, some of whom he
impressed with his proficiency at mathematics and logic. Others he put
off with his unsocial behavior. He met Maxwell, with whom he discussed pendulum theory
at the then-new Cavendish Laboratory. He also met the novelist Henry
James, who wrote to his brother William that Peirce had “too little art
of making himself agreeable.”
Pendulums involved little new theory.
Still, Peirce found a way to be original, applying his logical and
mathematical knowledge to study systematic errors due to the pendulum’s
mount. He developed a theory of such effects, showed that they explained various measurement discrepancies and errors, and designed an improved instrument. 5 5.
V. Lenzen, R. Multhauf, US National Museum Bulletin 240,
Contributions from the Museum of History and Technology, paper 44, Smithsonian Institution, Washington, DC (1965), p. 301.
Four identical pendulums of Peirce’s design were later constructed by
the Survey. The Smithsonian Institution has three of them (see the photo on page 40).
Peirce
reported his findings in 1875 to the Special Committee on the Pendulum
of the IGA in Paris. That made him “the first invited American
participant in the committee meetings of an international scientific
association.” 6 6.
M. Fisch, in Writings of Charles S. Peirce: A
Chronological Edition, Indiana U. Press,
Bloomington (1982–2009), vol.
3, p. xxv. On his return in August 1876, he brought a brass meter standard for calibrating American standards. They were numbered, and the one he brought was “no. 49.”
That
fall, after suffering a major nervous breakdown—the first of more than
half a dozen—Peirce moved to New York City to work on both gravimetrics
and spectroscopy
at the Stevens Institute of Technology in nearby Hoboken, New Jersey.
Zina remained in Cambridge, effectively ending their relationship.
Peirce soon took up with a woman named Juliette Pourtalai, conducting
the affair “far less discretely than the times demanded.” 7 7.
N. Houser, in ref. 6, vol. 4, p. xxii.
The gravimetric work left Peirce with a strong sense of the international character of the science.
As he wrote, “The value of gravity-determinations depends upon their
being bound together, each with all the others which have been made
anywhere upon the earth. … Geodesy is the one science the successful prosecution of which absolutely depends upon international solidarity.” 8 8.
C. Peirce, in ref. 6, vol. 4, p. 81.
Peirce’s spectroscopic work at Stevens initiated what would be the first measurement to tie the meter to a wavelength. The principle was simple, involving two measurements. One was to determine the angle of deviation of a ray of light passing through a diffraction grating, the other to establish the spacing of the grating lines. The familiar relation between spacing, bending angle, and wavelength then would connect the wavelength with the meter.
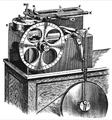
The engine built
by Lews Rutherfurd in the 1860s to rule lines for diffraction
gratings. Powered by flowing water, the engine’s wheels and gears
continually moved the grating’s platform (E) under the
diamond-tipped stylus (M) that scribed the lines. After a
line was completed, the micrometer screw (D) advanced the substrate
laterally by precisely the desired line spacing.
Peirce’s
motivation was the weakness of artifact standards. “Metallic bars used
as standards of length having more than once been found to have changed
their lengths in the course of years,” one of Peirce’s unpublished
manuscripts begins, “three different means of measuring such changes have been suggested.” 9 9.
C. Peirce, in ref. 6, vol. 4, p. 269.
One, carried out by two French scientists for the IGA, had been to rule
a meter on a bottle and fill it with water up to the rule. Any
expansion or contraction of the ruled meter over time (at the reference
temperature) would be signaled by a change in the mass of the water
needed to refill the bottle. A second means was to compare a length
standard with a seconds pendulum at a specified location. That idea had
been touted for a century, tried by the French revolutionary regime, and
enacted by the British in the Imperial Weights and Measures
Act of 1824. But when the Houses of Parliament burned in 1834,
destroying the existing artifact standards, the pendulum method proved
unreliable. The third method—Peirce’s proposal—was that “the standard
length may be compared with that of a wave of light identified by a line in the solar spectrum.”
The
proposal was not without problems. It involved “the assumption that the
wave-lengths of light are of a constant value,” Peirce wrote in 1879.
That was shortly before the Michelson-Morley experiments, and he was
worried about possible ether effects: “[T]here may be a variation in wavelengths if the ether of space, through which the solar system is travelling, has different degrees of density.” Astutely he added, “As yet we are not informed of such variation.” 10 10.
C. Peirce, in ref. 6, vol. 4, p. 4.
Peirce worked on this project for several years after his arrival at Stevens. The idea was based on the relation
between the wavelength λ, the line spacing d of the diffraction grating, the bending angle tH, and the order n of the diffraction pattern. Success depended on the gratings. They had become indispensable, having replaced prisms as precision instruments in spectroscopy and optics. “No single tool,” wrote MIT spectroscopy pioneer George Harrison in 1949, “has contributed more to the progress of modern physics than the diffraction grating.” 11 11.
G. R. Harrison, J. Opt. Soc. Am. 39, 413 (1949). https://doi.org/10.1364/JOSA.39.000413
In the 1870s the foremost—virtually the only—ruler of gratings was Lewis Rutherfurd (1816–92). An independently wealthy amateur astronomer and instrument maker, Rutherfurd had constructed an observatory in the garden of his home at the corner of 11th Street and Second Avenue in New York City. 12 12.
D. Warner, Technol. Cult. 12, 190 (1971). https://doi.org/10.2307/3102525 He was interested in solar photography and built a micrometer to measure
his solar photographs. Peirce used the micrometer to calibrate
centimeter scales for his pendulums. Rutherfurd became interested in spectroscopy
when Robert Bunsen and Gustav Kirchhoff made their startling assertion
in 1859 that spectra were fingerprints of chemical elements. Before
turning to diffraction gratings, Rutherfurd used prisms. In 1867, faced with the difficult task of ruling gratings before electric motors were available, he built an ingenious machine to rule gratings
on blanks made of glass or speculum, a copper-tin alloy. The engine was
driven by a turbine powered by water from city pipes. It used a diamond stylus and a micrometer screw to advance the blanks (see the figure above).
The gratings were treated with great care. About 4 cm wide, they were signed, dated, and inscribed with information about the lines
per inch—and kept in carved lacquer boxes of the sort made for
daguerreotypes. Several are now in the Smithsonian collection (see the figure above). Because Rutherfurd gave away the gratings at cost, he became quite popular among spectroscopists.
When Peirce came calling, Rutherfurd’s engine had a wheel with 360 teeth on its circumference able to rule 6808 lines per centimeter. Applying his usual care, Peirce noted imperfections in the grating: The stylus left a burr on one side of each line, which he found a way to remove. Rutherfurd’s work, he wrote, at last made it practical to think about measuring
“a wave length to one-millionth part of its own length.” Peirce
considered Rutherfurd’s contribution so essential that one unpublished
manuscript lists him as a coauthor.
Unfortunately,
declining health soon cut into Rutherfurd’s time. Interruptions ensued.
Peirce quarreled with Stevens colleagues and, in a tantrum, threatened
to quit the Survey. He was wooed back by Carlile Patterson, who had
replaced Charles’s father as superintendent. Then in September 1877,
Peirce left for Europe
for two months to join sessions of the fifth general conference of the
IGA. His formal presentation at the conference would be the first ever
by a US scientific agency at a meeting of an international scientific association. 6 6.
M. Fisch, in Writings of Charles S. Peirce: A
Chronological Edition, Indiana U. Press,
Bloomington (1982–2009), vol.
3, p. xxv.
On
shipboard, isolated from troubles, Peirce wrote an essay on scientific
method, “How to Make our Ideas Clear,” and he translated “The Fixation
of Belief,” which he had originally written in French. Those essays,
plus another four written shortly after and published as “Illustrations
of the Logic of Science,” are seminal in Peirce’s thought, articulating
the basic principles of the first phase of his pragmatic philosophy.
They betray the influence of his scientific work and his metrological
experiences in particular, which allowed him to appreciate features of science
that eluded—and still elude—those with a more formalistic outlook.
Scientists inherit tools, hypotheses, and experiences from predecessors,
Peirce argued. It doesn’t matter if these are imperfect, because science
is a fallible process in which a community of inquirers corrects errors
in ongoing revision. Knowledge grows, not in a staccato-like way in
which one representation replaces another, but rather in a continuously
expanding process in which a concept’s meaning is not an abstraction or
picture but the total of its effects on the world.
In those essays, Peirce developed a version of pragmatism different from that of his friend William James. Peirce approached science
as a matter not of solitary scholars confronting puzzles in private, as
James had, but of networks of competent people working in networks of
labs in an inherently public enterprise. Peirce also appreciated the
“economy of research.” An important part of science,
he argued, is maximizing the resources of available “money, time,
thought, and energy” when deciding what to work on. He realized that
there is no such thing as absolute precision. “Dealing as they do with
matters of measurement, [physicists]
hardly conceive it possible that the absolute truth should ever be
reached, and therefore instead of asking whether a proposition is true
or false, they ask how great its error is.” 13 13.
C. Peirce, in ref. 6, vol. 4, p. 241.
On his return to Stevens, Peirce continued his work of tying the meter to light. He measured the angular displacement of the image of a slit by a grating. Then, using a “comparator” that he had built, he compared the grating’s line spacing with the units of a glass decimeter he had calibrated using his no. 49 standard meter. He was, in effect, calibrating the grating spacing in units of wavelength.
Peirce now found that an obstacle to greater resolution was the appearance of “ghosts,” faint lines that appear on either side of principal spectral lines. They were clearly unreal because they only appeared in spectra created by gratings and never by prisms. Ghosts were created by tiny imperfections in the micrometer screws that had ruled the grating. But just as he had done with pendulums, Peirce treated the imperfections as opportunities: He measured them, developed a theory, and applied it to correct the measurements. With those adjustments, Peirce tried measuring a line Kirchhoff had identified near 5623 Å. But he ran into several sources of error, among them the thermal expansion coefficient of the glass grating and the quality of his thermometer.
Peirce published a brief progress report, “Note on the Progress
of Experiments for comparing a Wave-length with a Metre,” in the July
1879 issue of the American Journal of Science. “As soon as [the reduction of the errors and various calibrations are] done,” he wrote, “a metre will have been compared with a wave-length.”
Although Peirce had made too many enemies to get the physics chairmanship at Hopkins, the university did invite him to lecture
in logic. Even the best students—among them John Dewey, soon to be a
fellow pragmatist philosopher—found him difficult to understand. But
they did find him dazzlingly facile at many things. For instance, he
could simultaneously write on the blackboard a mathematical problem with
the right hand and its solution with the left.
Peirce kept improving his wavelength measurement, wrote a brief report in Nature (1881) entitled “Width of Mr. Rutherfurd’s Rulings,” made a report to the US superintendent of weights and measures
on the work, and began a summary, “Comparison of the Metre with a
Wave-Length of Light.” But the summary, like so much of Peirce’s work,
remained unpublished.
His life, too, was
unraveling. His father’s death in 1880 removed one protector;
Patterson’s death the following year removed another. Benjamin had tried
to groom Charles to take over the Survey, but the son was too
unpopular. Patterson was succeeded as superintendent by Hilgard, who was
incompetent, uninterested in research, and impatient with overcommitted
people like Peirce.
At Hopkins, Peirce
initiated an ugly quarrel with a visiting math professor over priority
for some discovery. He divorced Zina in 1883 and married Juliette a few
days later. Though he and Zina had been separated for seven years,
colleagues found such haste scandalous. Peirce fought with his cook, who
sued him for assault with a brick. “I have lately been offending people
everywhere,” Peirce wrote to Hopkins president Daniel Gilman in 1883.
Unfortunately, the offended parties included the Hopkins trustees, who
dismissed Peirce in 1884.
For a few
years, he continued to work with Hopkins students outside the classroom
and kept his assistantship at the Survey. From October 1884 to February
1885 he headed the Survey’s Office of Weights and Measures.
But he became ensnared in a scandal—for once not of his own making—and
subjected to congressional investigation triggered by charges of
drunkenness and other misconduct that others had lodged against Hilgard.
Peirce’s
attempt to use an optical wavelength of light as an absolute length
standard inspired other attempts. One was at Hopkins, where Rowland
began to manufacture gratings
superior to Rutherfurd’s. Rowland’s work helped make the university a
center of optical research from the 1880s to World War II. His student
Louis Bell achieved a spectroscopic accuracy of a part in 200 000 with Rowland gratings. 14 14.
L. Bell, Am. J. Sci. 33, 167 (1887).
Another attempt was made at the Case School of Applied Science in Cleveland, Ohio, where Michelson had read Peirce’s publications. Michelson realized that the interferometer he and Morley had developed and were just then using to detect ether drift could also be used to make precise wavelength measurements. In June 1887, after getting initial results in their epochal experiment on the speed of light, Michelson and Morley conducted preliminary spectroscopic metrology measurements. Their paper “On a Method of making the Wave-length of Sodium Light the actual and practical Standard of Length” begins, “The first actual attempt to make the wave-length of sodium light a standard of length was made by Peirce.” 15 15.
A. Michelson, and E. Morley, Am. J. Sci. 34, 427 (1887). But, they pointed out, Peirce’s measurements, “which have not as yet been published” (and never would be), had many systematic errors.
Those errors were overcome by the Michelson-Morley interferometer, which split a beam, sent its two parts along different paths, and recombined them to create an interference pattern. At one mirror Michelson and Morley installed a micrometer to move the mirror
a precise distance while counting interference fringes. By using a
micrometer and fringes where Peirce had simply used a ruler, they
greatly reduced measurement errors. Their work dramatically illustrated the limitations of Peirce’s approach—but also its revolutionary potential.
Benjamin
Gould, then the American member of the BIPM, relayed word of the
Michelson-Morley length-standard work to BIPM bureau’s director René
Benoît, who invited Michelson to work there on the idea. Michelson
arrived at Sèvres in 1892 and measured the red line of cadmium to a part in 10 million. But the formal redefinition of the meter—in terms of a krypton-86 line—would have to wait until 1960.
In
1887 Peirce and Juliette moved to Milford, Pennsylvania, where they
purchased an estate he named Arisbe after an ancient Greek town.
Approaching 50, he left most of his scientific work behind, though he
did occasionally dabble in metrology. Stimulated by Michelson, he briefly considered returning to measuring
wavelengths. In a review of a book by Edward Noel, an amateur
metrological reformer and opponent of the metric system, Peirce ventured
into social aspects of metrology:
He was unenthusiastic about Noel’s antimetric ideas, but his pragmatic
instincts made him doubt the prompt acceptance of that supposedly
rational system. Considering the way America was parceled into acres and
lots, and the way all machinery consisted of parts liable to break or
wear out “and must be replaced by another of the same gauge almost to a
thousandth of an inch,” Peirce wrote, “[e]very measure
in all this apparatus, every diameter of a roll or wheel, every
bearing, every screw-thread, is some multiple or aliquot part of an
English inch, and this must hold that inch with us, at least until the Socialists, in the course of another century or two, shall, perhaps, have given us a strong-handed government.” 16 16.
C. Peirce,The Nation, 27 February 1890, p. 184.
Peirce was now evolving from logician and laboratory-minded philosopher of science into a thinker who incorporated insights about science into a comprehensive theory of inquiry. That theory expressed a deep appreciation for the role of chaos and chance, with which he had become familiar through metrology
and thermodynamics. Nature is stochastic all the way down. Even in the
most refined and advanced inquiry, at some level we are still ultimately
in the position of upholsterers cutting curtains for windows.
Though
Peirce matured as an original philosopher in his years of “retirement,”
he never mastered the art of making himself agreeable. He grew
increasingly estranged from friends and from opportunities for
livelihood. Having been forced to resign from the Coast and Geodetic
Survey in 1891, Peirce became so destitute that James appealed to
friends on his behalf. In 1897 James wrote to James Cattell, the
publisher of Science , “Glad to receive $10 for Peirce, who has few friends.”
In 1899 Peirce sought unsuccessfully to become inspector of standards for the Survey’s Office of Weights and Measures. He had to watch from a distance when the US
Bureau of Standards, for which he had agitated, was created in 1901.
The fund established by James supported Peirce for the rest of his life.
He died at Arisbe in 1914.
Thanks to David Dilworth for discussions of Peirce, and to Harold Metcalf for discussions of spectrometry.
- 1. V. Lenzen, Proc. Am. Phil. Soc. 109, 29 (1965). Google Scholar
- 2. J. Brent, Charles Sanders Peirce: A Life, Indiana U. Press, Bloomington (1998). Google Scholar
- 3. I. Morus, When Physics Became King, U. Chicago Press, Chicago (2005). Google ScholarCrossref
- 4. S. Schaffer, in Victorian Science in Context, B. Lightman, ed., U. Chicago Press, Chicago (1997), p. 438. Google Scholar
- 5. V. Lenzen, R. Multhauf, US National Museum Bulletin 240, Contributions from the Museum of History and Technology, paper 44, Smithsonian Institution, Washington, DC (1965), p. 301. Google Scholar
- 6. M. Fisch, in Writings of Charles S. Peirce: A Chronological Edition, Indiana U. Press, Bloomington (1982–2009), vol. 3, p. xxv. Google Scholar
- 7. N. Houser, in ref. 6, vol. 4, p. xxii. Google Scholar
- 8. C. Peirce, in ref. 6, vol. 4, p. 81. Google Scholar
- 9. C. Peirce, in ref. 6, vol. 4, p. 269. Google Scholar
- 10. C. Peirce, in ref. 6, vol. 4, p. 4. Google Scholar
- 11. G. R. Harrison, J. Opt. Soc. Am. 39, 413 (1949). https://doi.org/10.1364/JOSA.39.000413 , Google ScholarCrossref
- 12. D. Warner, Technol. Cult. 12, 190 (1971). https://doi.org/10.2307/3102525 , Google ScholarCrossref
- 13. C. Peirce, in ref. 6, vol. 4, p. 241. Google Scholar
- 14. L. Bell, Am. J. Sci. 33, 167 (1887). Google ScholarCrossref
- 15. A. Michelson, and E. Morley, Am. J. Sci. 34, 427 (1887). Google ScholarCrossref
- 16. C. Peirce,The Nation, 27 February 1890, p. 184. Google Scholar
- © 2009 American Institute of Physics.
Article Metrics
Altmetric
Please Note: The number of views
represents the full text views from December 2016 to date. Article
views prior to December 2016 are not included.